As we confront the ubiquity of generative AI, the questions we need to ask ourselves as educators are: does thinking take place in our classrooms? At what level? And has technology shifted the educative goals in our classroom?
According to famed US developmental psychologist Howard Gardner, in 5 Minds for the Future, the first type of mind that people will need to thrive is a “disciplined” mind. To me, that means students must do more than accumulate knowledge and procedural-based problem-solving skills. They must also, in my classroom at least, learn to think like a physicist. So, as educators, we have to provide meaningful learning opportunities for them to do so.
As author and associate professor in physics Chad Orzel puts it, thinking like a physicist is “a very particular approach to problem-solving that involves abstracting away a lot of complication to get to the simplest possible model that captures the essential elements of the problem”. To paraphrase Einstein: “Make it as simple as possible, but not simpler.”
To allow my students’ thinking to take place, I need to observe and facilitate – that is, be a “guide on the side” rather than a “sage on the stage”, so students have sufficient space to think like physicists.
- Resource collection: Teaching critical thinking
- How to humanise engineering education and why we must
- Striving for imperfection: introducing randomness into virtual experiments
In the rotational mechanics class that I share here, I started with a complex inquiry-based learning (IBL) task that both challenges students and motivates them to persevere. It is the process as well as reflecting on that struggle which helps them inch closer to developing their “disciplinary mind”. In carefully designing such lessons, I always have two practical constraints to resolve:
- different readiness levels of students
- limited class time.
To address the first, I provide scaffolding so less-ready students can appreciate the abstract physics concepts at work without feeling lost. For the second, I minimise the class time that students spend on lower-order thinking work (such as data collection or plotting graphs) so they have time to focus on analysis and evaluating work.
Challenging and motivating students with an IBL-based exercise
I would like to use one such lesson to illustrate in practice how I overcame these constraints.
Ignite the inquiry process
To start the lesson, which introduces the concept of rotational inertia, I introduce the investigative task: students are challenged to find k (which refers to a physical quantity related to an object’s rotational inertia) of a tin can rolling along a plane (see below).
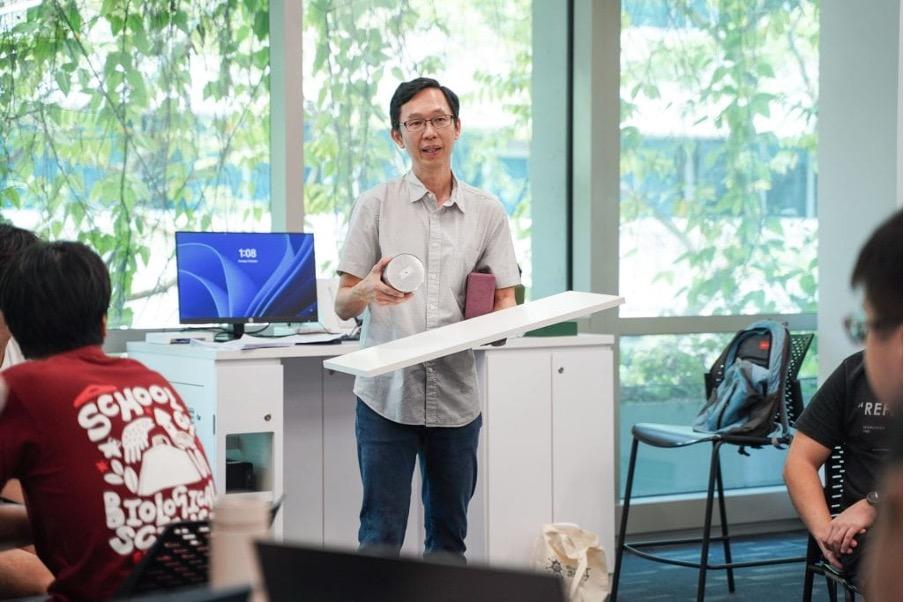
Connect and hypothesise
To scaffold the inquiry process, I ask students to work in pairs. A worksheet guides them to make connections with prior learning, and apply that theoretical knowledge to hypothesise the relationship between rotational inertia and an object’s physical properties. This mirrors the theoretical groundwork that physicists undertake in a collaborative context. The pair work and worksheet support students who may not have a strong background in physics.
Investigate
In pairs, students video the rotational movement using their smartphones. Then, they upload the video into Tracker, an application that plots the movement into a graphical representation. This plunges the students straight into the analytical and evaluative-thinking process, minimising time spent on lower-order laboratory work. The students can also appreciate the complexity (and messiness) of working with real-world data. Both the troubleshooting process to clean the real-world data for analysis, and the augmentation and modification of the learning process through technology reflect the data collection and analysis practices of physicists.
Present and reflect
Finally, through facilitative questioning, students are guided to make their thinking visible, evaluating their results against their theoretical predictions. They reflect independently on what worked and what didn’t in their investigation. This practice is a key part of the scientific process, driving advancements and deepening understanding.
One student said: “It was quite fun to learn how to use a new software, and felt rewarding when our group was able to figure out how to use the software to obtain our required values.
“It was frustrating when we forgot to calibrate our frame rate, but we ultimately were able to work it out to recalibrate the t axis and obtain the right acm, which was highly satisfying.”
Bridging the gap: from learning physics to being a physicist
Understanding physics is not just about absorbing information but about learning how to think through problems, develop hypotheses, collect and analyse data, and reflect on findings, as the lesson illustrates. Learning to be a physicist fosters universally valuable skills such as critical thinking, problem-solving and analytical reasoning. This mindset, characterised by curiosity, rigour and persistence, equips students to tackle complex problems, challenge assumptions and make evidence-based decisions, preparing them for future challenges in their professional and personal lives.
Moreover, the scientific inquiry process that is integral to physics mirrors the process of lifelong learning. It encourages comfort with uncertainty, a willingness to test and revise ideas based on evidence, and an openness to continuous learning. These attributes are beneficial for everyone, not just physics majors or budding scientists. Thus, the approach of “learning to be a physicist” has broader implications, fostering a mindset and skillset that can serve students well in any field and any aspect of life.
Elevating inquiry-based learning with technology
Technology, when combined with an IBL approach, can transform the learning experience, reshaping students’ pathway towards achieving their learning objectives.
For instance, students could use digital databases for research as a starting point, but then deploy text-analysis tools or data-mining software to explore these resources further. This encourages them to move beyond passively consuming information to actively generating new insights, a transformation that aligns with the modification level of the substitution, augmentation, modification and redefinition (SAMR) model for implementing technology and the principles of IBL.
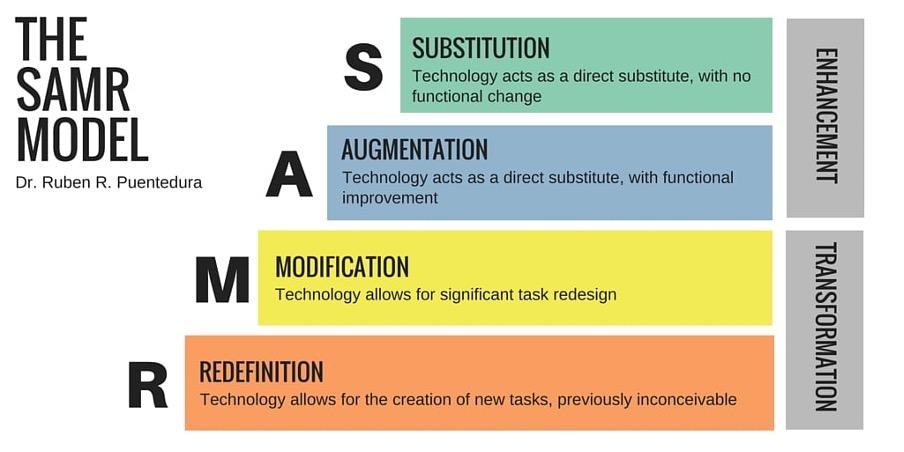
Students could also use technology to create innovative presentations. They could use video-editing software to produce mini-documentaries or animated explainer videos, actively engaging with the content in a creative way that aligns with IBL’s emphasis on communicating new insights.
The critical reflection stage, an important part of the IBL cycle, can also be enriched with technology. Students could create video reflections or vlogs, sharing their learning journey in a personal and engaging format. This not only promotes deeper reflection but also helps to foster a community of learners.
By integrating these modification-level uses of technology and the principles of IBL into teaching, we can enhance student engagement, foster critical thinking, and provide innovative ways for students to demonstrate their understanding.
Teck Seng Koh is a senior lecturer and assistant chair in education and pedagogy development in the School of Physical and Mathematical Sciences at Nanyang Technological University, Singapore.
This is an edited version of the blog post “Learning to be a physicist: technology-enhanced inquiry-based lesson to develop disciplinary thinking” first published by the NTU Institute for Pedagogical Innovation, Research and Excellence.
If you would like advice and insight from academics and university staff delivered direct to your inbox each week, sign up for the Campus newsletter.
comment