How do educators put real-world problems in the minds of students? Visits from industry experts and exposure to prototypes are effective, especially in medical and engineering fields. But I have also been known to ask them to interview their grandparents to find out what exactly an ageing population might find useful in their healthcare products.
I tell my students: “Who are your customers? Your grandparents. Talk to them, really identify key challenges they are facing.” This encourages the future engineers and materials scientists I teach to formulate a design-thinking approach from day one.
My approach to teaching has evolved – from content-focused to a “triple helix” model that integrates instructor-led learning, experiential learning and problem-driven approaches – in the 16 years since I began teaching at Nanyang Technological University.
- Lessons from an interdisciplinary community engagement course for engineers
- How to humanise engineering education and why we must
- ‘We should focus on the effect of the technology rather than the technology itself’
Back then, my heavily content-focused method was underpinned by my own nervousness as a new professor. I was trying to teach a lot of material. The traditional, now-outdated model typically involved detailed lecture slides covering every technical concept and theory I aimed to convey. Following the lecture, standard tutorial sessions and assessments left little interactivity or hands-on learning.
The instructor-centred approach was exhausting for me and my students. Worse still, it also failed to bridge the gap between academic knowledge and real-world application.
Triple helix and a flipped classroom
As my teaching progressed, I realised that engineering education demands more than just top-down theoretical knowledge. It also requires students to develop practical skills, critical thinking and industry awareness. So, about nine years ago, I began to develop a new model of pedagogy for my courses.
This didn’t mean completely abandoning instructor-led teaching. Rather, it became one part of a system I refer to as the “triple helix” (see below).
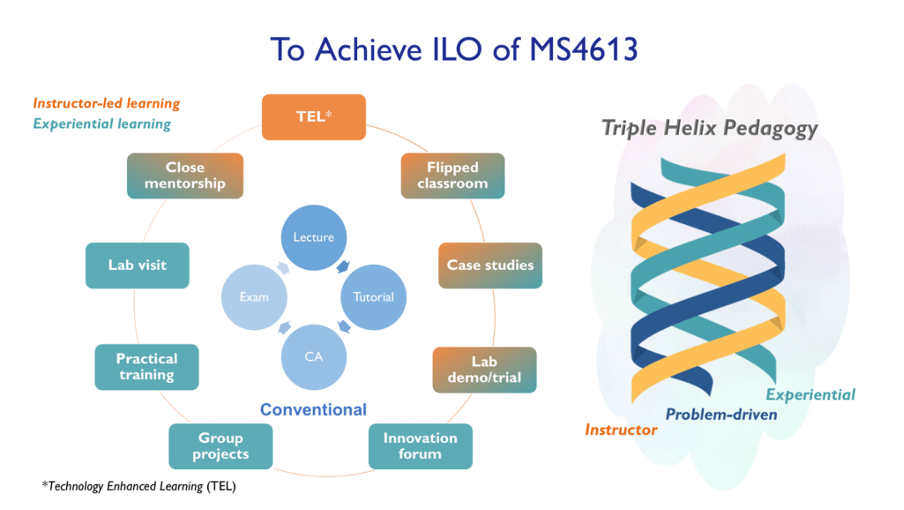
This pedagogical framework transforms conventional teaching elements through technology enhanced learning, integrating instructor-led and experiential learning with a problem-driven mindset. Instructors count as only one part of the system.
The “flipped classroom” enables all parts of the triple helix; students learn foundational concepts before class, which frees up in-class time for deeper engagement. That may take place through discussions, hands-on lab work, real-world case studies and problem-solving exercises.
In the case of my MS4613 Wearable Sensors for Healthcare course, pre-class lectures, reading materials and online exercises cover fundamental topics such as sensor materials, fabrication techniques and healthcare applications. In class, students then apply their knowledge through group projects, technical problem-solving and prototype development, all of which they engage in through a cleanroom facility environment.
Shifting students from a passive note-taking role to active learning while offering them one-to-one interactions with instructors and mentors means they can better retain knowledge and an understanding of what it means to apply the concepts they learn.
Real-life problem-solving
By Year Four, students are expected to design, prototype and present early-stage engineering designs. Student teams develop innovative healthcare solutions, demonstrating their ability to translate theoretical knowledge into working prototypes, which helps them connect what they learned in previous semesters to real applications.
As educators we can draw parallels between classroom experience, and our own work as scientists (as an engineer in my case).
For example, our lab recently made a discovery about the unique properties of spider silk. The team found the material had an ability to become soft and pliable when exposed to water, and that materials with similar properties could be used to bridge the mismatch between rigid electronics and soft biological tissues, like human skin and organs.
This real-world connection is the same reason students should interview their grandparents – to understand how discoveries in the lab might be applied in society.
Mentors at the ready
The triple helix process also opens the door for mentorship. Smaller student-to-teacher-assistant ratios – of, for example, around five to one – ensure that plenty of on-site advisers are available for consultation.
Smaller groups of 15 to 20 students also allow for more detailed discussion during presentations. When we increased classes to 30 students, we found it harder to maintain the same depth of mentoring and project guidance.
Inviting industry professionals to speak to engineering classes helps expand students’ problem-driven mindset. In these encounters, students see different perspectives on their projects. Questions about manufacturing costs or market feasibility push them to think beyond pure technical specifications; practical industry feedback can lead to design refinements.
Innovation forums also create natural opportunities for students to articulate their ideas to non-specialist audiences. Presenting prototypes to industry representatives requires clear communication of both technical concepts and potential applications – skills essential for engineering careers.
If a primary teaching goal is for students to be “industry ready”, this means continual refinement of our courses, year after year, to keep pace. For example, I may have once merely talked about artificial intelligence, but now we are living with AI. As a result, case studies have changed and so has the way I have students present their work. I might ask for video presentations rather than written ones.
An investment in the future
Overall, I’ve realised a university is not just there to teach content; it’s also to inspire. And my number one job is not research, it’s education. It’s about enabling our next-generation students. This is the main product of the university, and research is basically a way to educate our students.
To further elucidate this point, I think of a Chinese proverb: It takes 10 years to grow a tree, but a hundred years to cultivate a person.
The triple helix model means that all elements of an engineering education – instructor-led and experiential learning and problem-solving opportunities – bind together to form a strong foundation. With this investment of time, mentorship and deep learning, students will branch out into their own discoveries and innovations.
Chen Xiaodong is a professor in materials science and engineering, a distinguished university professor and deputy director of the Institute for Digital Molecular Analytics and Science at Nanyang Technological University, Singapore.
This is an edited version of the blog post “Seeking real-world impact through pedagogy”, which was first published by the NTU Institute for Pedagogical Innovation, Research and Excellence.
If you would like advice and insight from academics and university staff delivered direct to your inbox each week, sign up for the Campus newsletter.
comment